How valence electrons determine molecular shape?
To predict molecular shape, we usually use a theory called the valence shell electron pair (VSEPR) repulsion theory. This theory is based on the idea that valence electrons in a molecule tend to repel each other to create more space around them. Let’s use methane molecule to further explain this.
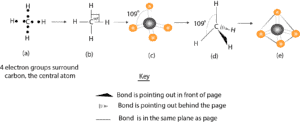
Molecular shape of methane
Model (a) describes the Lewis dot structure for methane. Recall that the dots represent the electrons. Model (b) is similar to model (a), except that we have drawn lines to represent the four bonding pairs of electrons. Since electrons carry a negative charge, the region around each bonding pair of electrons will be concentrated with a negative charge. And because like charges repel, the electrons in the four bonds of methane will repel one another.
How can these electrons possibly minimize this repulsion?
To minimize the repulsion, the electrons in the four bonds must arrange in a three-dimensional space such that they can open the H—C—H bond angles beyond 90°. If these bonding electrons arrange such that they open the H—C –H bond angle up to 109°, then we will get an arrangement in (c) represented by the ball-and-stick model. But since the ball-and-stick model is bulky on paper, we can describe the three-dimensionality of molecules by drawing the model represented in (d). Notice that in (d) the solid wedge means that the bond is sticking out from the paper and pointing toward you, while the dashed wedge means that the bond is sticking out behind the paper and pointing away from you and the paper. The lines mean that the bonds are in the plane of the paper.
If we consider the four bonds to point away from the center atom (carbon) towards the four corners of a tetrahedron (a figure that consists of 4 equilateral triangles put together), we will get a representation similar to the one in (d). When the bonding electrons are arranged this way, they no longer repel one another so much, and it’s the best arrangement we can possibly get that increases the stability of the molecule, but reduces the repulsion between the bonding electrons. As a result of this arrangement, we usually say methane molecule has a tetrahedral shape.
What’s the shape of a water molecule?
A water molecule has four electron groups around the oxygen atom. Of these four electron groups, two are lone pairs of electrons and two are bonding pairs of electrons. Figure (a) below shows the Lewis dot diagram of water molecule, while figure (b) shows its arrangement in three-dimensional space.
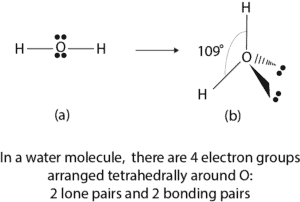
From figure (b), we may be tempted to say that water has a tetrahedral shape because it has four electron groups surrounding it. But that’s not right. Usually we include the lone pairs to help us determine how all the electron groups are arranged around the atom. After that, lone pairs are excluded when we try to describe the shape of the molecule. If we exclude the lone pairs on the oxygen atom in figure (b), we can describe its shape as bent or V-shaped depending on how you look at it.
What’s the shape of carbon dioxide molecule?
From the figure below, if we count each double bond as a single bond, we can only have two electron groups about the carbon atom. Ignore the lone pairs of electrons on the oxygen atoms. Only electrons on the inner atoms can affect the shape of carbon dioxide molecule. Let’s use the following models to figure out the shape of carbon dioxide molecule.
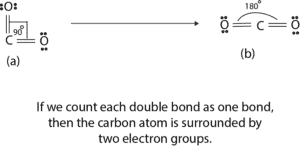
If the atoms arrange such that the O—C—O angle is 90°, there will be a stronger repulsion between the bonding pairs of electrons, making the molecule unstable. As a result, the best way the bonding electrons can minimize the repulsion between them is to arrange linearly such that they can be 180° apart as in (b). And when the atoms in CO2 arrange this way, we usually say carbon dioxide has a linear shape.
Why is the shape of a molecule important?
Molecular shape is important because the physical and chemical properties of molecules depend on it. Have you ever wondered why water behaves the way it does? For example, water can change its state from steam to liquid and to ice without changing its composition. To read why water does this, click here.